⇐ Previous Article — Table of Contents — Next Article ⇒
New Energy Times home page
2. The Emergence of an Incoherent Explanation for D-D "Cold Fusion"
By Steven B. Krivit
Abstract
In 1998, a group of researchers performed a LENR experiment that resulted in one of the most important findings of the field: a clear relationship between the measurements of excess heat and 4He. This finding helped to establish proof for the reality of nuclear production of both excess heat and 4He in LENR.
The only problem with the 1998 experiment was that the measured values of excess heat, expressed as MeVs per helium-4 atom, did not agree reasonably with the ~24MeV/4He prediction of the hypothesized D+D —> 4He + heat “cold fusion” reaction. It turned out, instead, to be 32MeV/4He.
In addition to the subgroup of LENR theorists who had developed models based on the hypothesized D+D —> 4He + ~24 MeV (heat) "cold fusion" reaction, some experimentalists were also convinced that LENR experiments produced ~24 MeV of heat per 4He atom. Had those experiments shown ~24Mev/4He, they would have helped to validate the theorists' models and the experimentalists’ hopes of LENR's being a "cold fusion" process.
Starting in 2000 and continuing in 2004, several members of this subgroup drew on older data, from a 1994 experiment, and applied inexplicable new assumptions, invented new concepts of metallurgy that conflicted with century-old knowledge, reinterpreted the 1994 experiment with radically different conclusions, and created the impression that the two experiments occurred in reverse order.
The result of this behavior was the birth of an incoherent hypothesis of helium retention and release that, if true, would have helped to explain the large discrepancies between D-D "cold fusion" theory predictions and the values of excess heat expressed as MeVs per helium-4 atom that were measured in the 1998 as well as the 1994 experiments.
Members of this subgroup presented the results of their effective re-analysis of the 1998 and 1994 experiments to the Department of Energy in 2004. However, they did not inform the DoE that it was not, in fact, a re-analysis. More important, their report was laden with assumptions and analyses having little to do with the actual data.
In June 2004, Italian collaborators of the American proponents of the DoE review performed a LENR experiment in which they, too, claimed to measure
MeVs per helium-4 atom, reasonably agreeing with the ~24MeV/4He prediction of the hypothesized D+D —> 4He + heat “cold fusion” reaction.
The report from this Italian group contains multiple illogical, incoherent representations. The article "Inexplicable Cold Fusion Claims From Italy" in this issue goes into them in depth.
The DoE and its reviewers most likely did not know about the underlying issues or realize that they were presented with a biased view of the LENR field from this subgroup.
However, for a number of reasons, the DoE and its reviewers didn't buy the idea that LENR was explained as a D-D "cold fusion" process. As a partial result, the official acceptance of the phenomena that many LENR researchers and supporters worldwide were hoping for did not occur.
Thus, the biggest opportunity that had been made available to researchers in this field in 15 years was wasted. The article “U.S. Department of Energy 2004 LENR Review—The Inside Story” in this issue explores the 2004 DoE LENR review in detail.
Through a careful review of the information presented to the DoE and the original report and data from the 1994 experiment, New Energy Times reveals how the process occurred. Many of the DoE reviewers had a sense that something was not quite right with the review paper presented to them by the review's proponents. This investigation lays those ambiguities bare.
However, all of these experiments clearly showed the production of helium-4 as a result of some nuclear process and showed that helium-4 production was, in fact, produced along with excess heat. The hypothesis of "cold fusion" was not proved; some other unspecified nuclear process, alluded to by the field's discoverers, Martin Fleischmann and Stanley Pons, is responsible for the anomalous observed experimental results.
Introduction
People who believe in the D-D "cold fusion" explanation of LENR claim that the proof is measured values of excess heat expressed as MeVs per helium-4 atom that agree with the prediction of the hypothesized D+D —> 4He + ~24MeV/4He (heat) “cold fusion” reaction.
As for other observed products of LENR, such as tritium, heavy-element transmutation products and MeV-energy charged particles, the D-D "cold fusion" hypothesis provides no answer. Furthermore, evidence of MeV-energy charged particles contradicts the D-D "cold fusion" hypothesis, which asserts that virtually all of the nuclear binding energy is released in the form of heat, with very little of the binding energy going into the kinetic energy of the alpha particle (4He). Finally, the subgroup that has been promoting the idea of the D-D "cold fusion" reaction implicitly assumes that the D-D "cold fusion" reaction is the only nuclear reaction taking place in the experimental system.
This report indicates that proof of the D+D —> 4He + ~24MeV/4He (heat) “cold fusion” reaction, at least in the most prominently reported LENR experiments, does not exist. This report does, however, affirm the very clear confirmation of the production of excess heat and production of helium-4 from LENR experiments and therefore confirms the validity of LENRs as nuclear phenomena.
In thermonuclear fusion, when two deuterium nuclei fuse, one of three branching possibilities results in the transmutation of the deuterium into a helium-4 nucleus, a gamma photon and energy.
The mass of the helium-4 atom is slightly less than the sum of the two deuterium nuclei. In accordance with Einstein's E=mc² equation, this mass deficit results in the release of nuclear binding energy — in this case, about 24 MeV.
Some LENR researchers considered attempts to measure experimental values of ~24 MeV very important because they thought such attempts would validate their hypothesis of a D-D “cold fusion” reaction in LENR experiments.
LENR theorist Scott Chubb, formerly with the Naval Research Laboratory, told this reporter in May 2008 why there is solid evidence to support the theory of "cold fusion."
“The proof is the 24 MeV! McKubre nailed it,” Chubb said.
Chubb was referring to an experiment performed by Michael McKubre et al. at SRI International in 1994.
McKubre and his colleagues have stated that this experiment came within 104% of 24 MeV, which equates to 24.75MeV. Oddly, they don't seem ever to have explicitly stated the MeV value. They stated only that it represented 104% of what they predicted based on the hypothesized D+D —> 4He + ~24MeV/4He (heat) “cold fusion” reaction.
The proof is the 24 MeV!
McKubre nailed it.
Scott Chubb
|
A number of LENR researchers in the last six years have promoted this 1994 experiment as the most accurate experimental evidence for proof of the hypothesized D+D —> 4He + ~24MeV/4He (heat) “cold fusion” reaction.
Curiously, people in the field (including this reporter) seemed to be largely unaware, until 2004, that results from this 1994 experiment had shown, as claimed by researchers from SRI and MIT, reasonable agreement with the hypothesized D+D “cold fusion” reaction. Considering the currently claimed significance of this experiment, that so few people knew about it before 2004 is odd.
In the course of this investigation, New Energy Times found that the first reported instance of this claim appears to have been in a 2000 paper, six years after the experiment occurred.
The paper, "The Emergence of a Coherent Explanation for Anomalies Observed in D/Pd and H/Pd Systems; Evidence for 4He and 3He Production," by
Michael McKubre, Francis Tanzella, Paolo Tripodi and Peter Hagelstein, was presented at the 8th International Conference on Cold Fusion in 2000 at Lerici, Italy. [2]
Why was this claim not reported until this time? The reason seems to be that only in 2000 did SRI and MIT researchers develop a new interpretation for the 1994 experiment.
A key aspect of this new interpretation required the development of the "helium retention hypothesis," which is explained in detail later in this article.
Why would the SRI and MIT researchers, who were claiming evidence and a theory for a D-D "cold fusion" reaction, reinterpret the results of a 1994 experiment six years later? Because in the 1994 experiment, the measured excess heat per helium-4 in experiment M4 did not agree with the prediction of the hypothesized D-D "cold fusion" reaction.
As the SRI and MIT researchers explained in 2000, though not coherently, the M4 experiment did not release all of the helium produced in the experiment into the gas phase, and therefore a portion of the produced helium was undercounted. Had this extra helium been released and counted, they claimed, the measured values of excess heat per helium-4 in 1994 would have agreed with the prediction of the hypothesized D-D "cold fusion" reaction.
This experiment, performed at SRI International, was a closed-cell electrolytic experiment with heavy water and a palladium rod. The SRI experiment is similar to the experiments performed by Fleischmann and Pons at the University of Utah in 1989, which quickly were labeled "cold fusion" by the media.
The results of the SRI experiment, called M4, were printed in a 379-page technical report by the project's sponsor, the Electric Power Research Institute, in June 1998. [1]
The conclusion of this 1998 report is significantly different from the perceptions
that exist in the minds of some LENR researchers about this experiment. They believe that the authors of this report definitively stated that they achieved very close agreement with the MeVs per helium-4 atom predicted by the hypothesized D+D —> 4He + ~24MeV/4He (heat) “cold fusion” reaction.
The report made no such statement. The report's conclusions, while providing for the possibility of such an agreement between prediction and experiment, stated that other explanations were also possible and that definitive statements could not be made from that experiment.
So how did the difference between the perception and the reality occur? The first reference found by New Energy Times is the 2000 paper by McKubre, theorist Peter Hagelstein, and colleagues.
But most people learned about the claimed agreement with the hypothesized D-D “cold fusion” reaction in 2004 when McKubre, Hagelstein and co-authors presented a LENR review paper to the DoE. They stated that one of four samples of helium from the 1994 experiment, known as M4, came within 104% of their prediction of the hypothesized D-D “cold fusion” reaction. They also claimed that this measurement represents the "most accurately determined [value] in this field." [3]
A Close Look at Experiment M4
Before we discuss SRI experiment M4, an explanation of how helium atoms are typically measured in LENR cells will be helpful.
A sample of the effluent gas is either withdrawn from the LENR cell or collected in a separate chamber. From the gas sample or from the collection chamber, researchers measure the concentration of helium, typically in parts per million of helium atoms. Based on the ppm concentration and the total volume of the cell, they can calculate the specific quantity of helium atoms.
During the course of the 1994 experiment, the researchers took four samples of helium from the LENR cell and performed measurements of each sample's concentration. These measurements (1.556 ppm, 1.661 ppm, 0.340 ppm and 2.077 ppm) are shown in slide 5 as S1M, S2M, S3M and S4M.
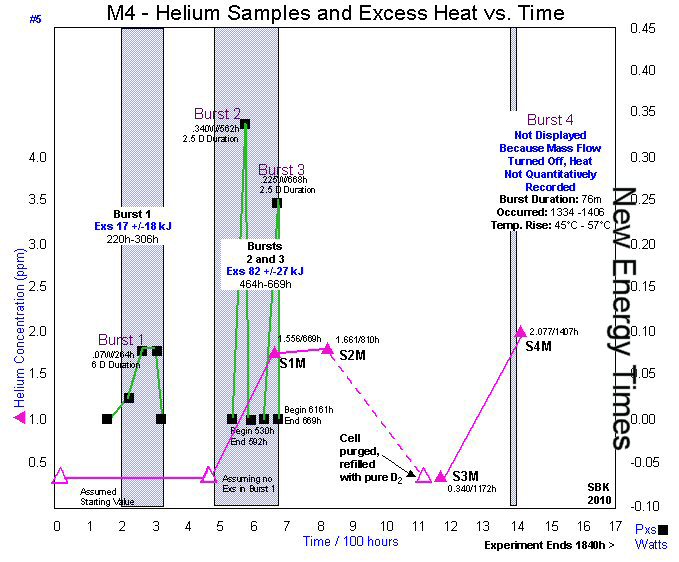
Figure 1 - Slide 5: M4 - Helium Samples and Excess Heat vs. Time
(Click here for a full-size image of slide 5, which will open in a new window.)
In their report, printed in 1998, the authors compared the measured helium
concentrations with the amount predicted from a D-D "cold fusion" reaction hypothesized by Melvin Miles and Ben Bush to produce 22.4MeV/4He atom.
Hagelstein was not part of the 1994 team. After an initial attempt in 1989 to explain the Fleischmann-Pons experiment as "fusion," he abandoned the attempt to explain LENR as a hypothesized D-D "cold fusion" reaction by 1993. [4]
He explained that he wanted to abandon the D-D "cold fusion" reaction hypothesis in order to avoid the Coulomb barrier problem as well as to find reactions that "more closely match the experimental observations."
Why he did another about-face in the late 1990s to re-embrace the D-D "cold fusion" reaction hypothesis is a mystery.
In comparing their results to the Miles/Bush prediction, the authors of the 1998 paper took into consideration a variety of dynamic factors in the system: cell volume, depleted gas volume from sampling, the addition of D2 to make up for the loss of depleted gas from sampling, and cell purging.
From these specific factors, they calculated a specific, dynamically changing level of predicted helium concentration for each of the first three helium samples. The 1998 authors did not attempt to suggest a predicted value for the fourth sample, presumably because it would have been meaningless considering the numerous uncertainties and cell perturbations during that period of the experiment.
These 1998 predicted values (3.76 ppm, 1.13 ppm, and 0.340 ppm) are shown in slide 3 by points S1P, S2P, and S3P, in blue.
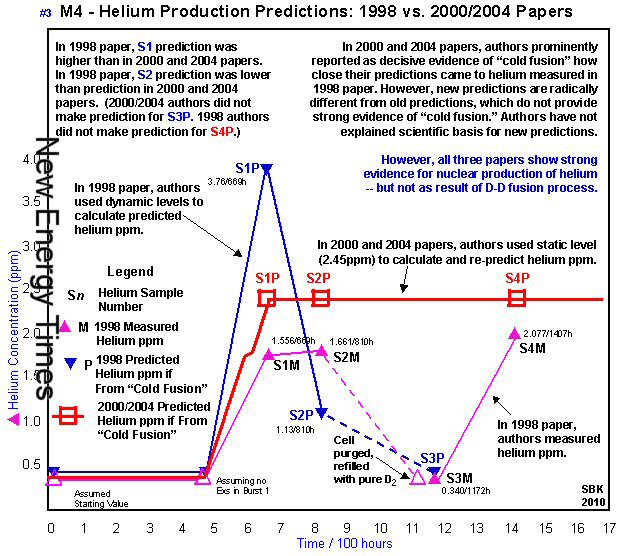
Figure 2 - Slide 3: M4 - Helium Production Predictions: 1998 vs. 2000/2004 Papers
(Click here for a full-size image of slide 3, which will open in a new window.)
In their analysis, the 1994 team members wrote that helium sample 1 was significantly lower (41%) than the predicted value. They wrote that sample 2 was significantly higher (147%) than the predicted value.
Slide 5 shows that researchers took helium sample 1 immediately following two significant bursts (No. 2 and No. 3) of excess heat. The 1998 report is forthright about an analytical weakness; The researchers do not have a helium sample between burst No. 1 and burst No. 2.
The 1998 authors did not state any possible excess heat from burst No. 1 because the value (17 +/-18 kJ) was too close to the detection limit.
Between sample 1 and sample 2, no excess heat was produced. Researchers perform two actions during this time. As shown in reference 3 of slide 4, at hour 740 they reversed polarity on the electrodes to run an anodic strip to clean to impurities from the cathode. The researchers generally ran the anodic strips for five to 10 minutes. When the cathode is stripped of the impurities and normal current is resumed, the cathode can continue absorbing even more deuterium and increasing the D/Pd loading density. At hour 758 (reference 4), the researchers performed a series of three six-hour heater power steps to verify the calorimeter calibration. The report does not explain why researchers decided to take sample 2 at the time they did.
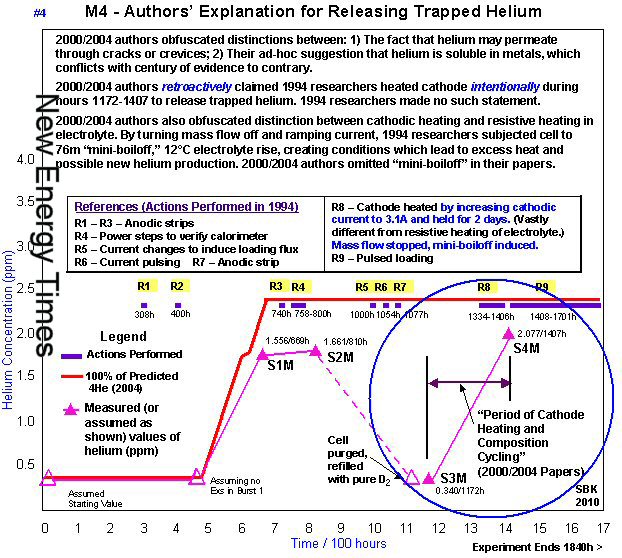
Figure 3 - Slide 4: M4 - Authors’ Explanation for Releasing Trapped Helium
(Click here for a full-size image of slide 4, which will open in a new window.)
After taking sample 2, the researchers performed three sets of actions (References 5-7) to induce deuterium flux, a factor known to promote excess heat, and another cathodic strip. But the cell produced no excess heat as a result of these three actions.
Without taking another helium sample, they purged the cell and refilled it with pure D2 gas. They did not explain why they decided to purge the cell. However, because their previous actions failed to elicit an excess-heat response, they may have decided to start fresh.
Toward the end of the purge/refill, they took their third helium sample, which showed a concentration of 0.34 ppm. This matched what they predicted based on a cell filled with fresh D2 gas.
Incoherent Explanations of Experimental Procedure and Purpose
Beginning at hour 1334, the experiment enters a period, between helium samples 3 and 4, in which the authors of the 2000 paper restated the intentions of the 1994 researchers and suggested the 1994 researchers made efforts to drive "trapped" helium out of the palladium. The significance of the trapped, or retained, helium hypothesis will be explained in just a moment.
Reference 8 on slide 4 provides a visual display of the actions that the 1994 researchers applied to the cell during this period (pg. 3-223 PDF pg. 351 and pg. 3-174 PDF pg. 302). The 1994 researchers described the steps as follows:
"Induce loading variation by switching the current on alternate measurement cycles between 3.1A cathodic and 0.001A anodic." |
"Cathode was ramped from 0.1 to 3.1A at 25mA/hour, held at 3.1A for ~2 days." |
"The cell was also subjected to 'mini-boiloff,' with the mass flow stopped for 76 minutes; during this time, the cell electrolyte temperature rose to 57 degrees C (from 45 degrees C)." |
These actions taken by the 1994 researchers are typical of those they would take either to achieve higher D/Pd loading ratios or to trigger excess heat.
However, these actions were redescribed by SRI and MIT authors in 2000/2004 papers. The authors stated that the 1994 researchers performed some or all of these actions in efforts to heat the cathode to release trapped helium.
But nowhere in the detailed, step-by-step explanations of the 76-day experiment did the authors of the 1998 paper state anything about a retained helium hypothesis, let alone mention efforts to drive the helium out of the palladium.
Then there's another problem. The authors also deliberately blurred the distinction between cathodic heating and resistive heating in an electrolyte. Heating a cathode by running current through it is precisely what causes the excess-heat effect. To suggest that this form of heating was unequivocally not contributing to a possible heat effect and "releasing dissolved helium" is unsupportable.
What is not stated at this point in the 1998 report but stated later at page 3-223 (PDF pg. 351) is that the 1994 researchers induced a short heat burst, a "mini boil-off," during this period by turning the mass flow off. The flow normally runs at 20 degrees C and therefore has a cooling effect on the cell, which works against the positive heat coefficient, which is known to enhance excess heat in LENR cells.
During the 76 minutes when they had the flow off, the researchers measured a 12-degree rise of electrolyte, from 45 degrees C to 57 degrees C. During this period, they also registered a rapid burst in the Pd/D loading.
The authors of the 1998 paper wrote, "We cannot rule out the possibility that 4He was sourced during the period between samples 3 and 4." On the other hand, the authors of the 2000/2004 papers failed to mention this heat burst.
Immediately following this 72-hour period, the researchers took the fourth helium sample and measured 2.077 ppm. They likely decided to take the sample at this time because they suspected the 76-minute mini boil-off caused a LENR excess-heat reaction and, quite possibly, new helium.
The 1998 authors did not speculate on possible excess heat for this burst because they did not have calorimetry mass flow data during this period. As well, they noted other calorimetric uncertainties during this time. Appropriately, without rigorous data for excess heat during this period, the authors of the 1994 work also did not speculate on a predicted value of helium with which to compare the helium sample 4. Nor is there any evidence that the 1998 authors had, as a working hypothesis, the idea of helium retention. The first appearance of this hypothesis in the paper is described in the analysis phase of the report, not in the experimental section.
The experiment continued past hour 1407, but there were no further periods of excess heat or helium samples taken.
Reprediction of Helium Values
For reasons not known to New Energy Times, the authors of the 2000 paper and the 2004 paper reinterpreted the 1998 data. None of the four authors of the 2000 paper returned e-mail inquiries asking for an explanation for the basis of the new values.
On Jan. 20, this reporter met with Francis Tanzella at SRI "on background." Over the course of a two and a half hour meeting, this reporter reviewed many aspects of experiment M4. Tanzella was cordial and very helpful. This reporter learned a few new details of the basics of Pd/D electrochemistry and confirmed the appearance of two minor typographical errors in one of the 1998 report's graphs.
Table 1: Helium predictions based on measured heat and a D-D "cold fusion" reaction for experiment M4. Experiment performed in 1994 and reported in 1998. Comparison between 1998 paper and 2000/2004 papers.
As shown in Table 1, the data reported in 1998 did not strongly agree with the prediction of a hypothesized D-D “cold fusion” reaction. In sample 1, researchers found 41% of the predicted amount of helium, had it been sourced from a D+D —> 4He + ~24MeV/4He (heat) “cold fusion” reaction. In sample 2, they found 147% of the predicted amount.
Sample 3 is a control. Researchers flushed the cell, filled it with pure D2 and measured 100% of what they would expect from those conditions. As mentioned above, the 1998 authors did not speculate on a predicted amount of helium in sample 4.
The 1998 authors did not use a static value — that is, a similar value of helium from which to predict for samples 1 and 2. The reason is that the gas volume changed between the two samples; they were correct to use dynamic values. The 1998 authors took this into account when dynamically calculating their predicted values of helium (41% and 147%). Slide 3 shows this graphically.
The D-D "Cold Fusion" Claim to the Department of Energy
Despite the numerous variances and inconsistencies between the original 1998 paper and the 2000/2004 papers, the authors of the 2004 paper made the following definitive statement to the Department of Energy about sample 4 from experiment M4: "The final integral mass balance yielded a value of 104± 10% of the expected value if the excess power in Figure 5 is due to a reaction of the sort D+D > 4He + ~ 23.8 MeV (heat)."
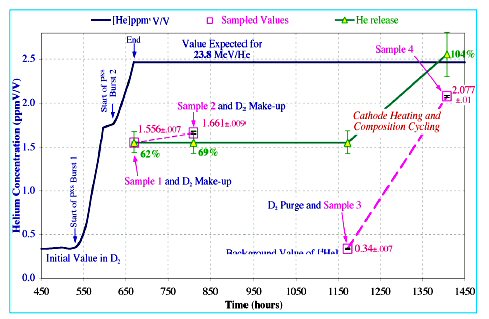
Figure 5 - Representation of helium growth from SRI experiment M4 in paper presented to the Department of Energy. Shows four samples of helium measurements. [3]
New Energy Times sent queries to all four authors of the 2000 paper in which most of these incoherent explanations first emerged. We asked them about the discrepancies between the 1998 paper and the 2000 paper.
We summarized the specific conditions that existed and the actions that occurred in the cell between helium sample 3 and sample 4. We asked them the following questions:
— Can you conceive of any way that the majority of the 2.077 ppm of helium [1] measured in sample 4 could have come from the heat bursts (No. 2 and No. 3) during 464h to 669h, and if so, can you please explain what that would be?
— Have any significant errors in the 1998 paper, specifically with regard to the predicted helium, ever been reported?
— Can you explain the changes in the predicted values of helium as written in the 2000 paper from the original predicted values shown in the 1998 paper?
|
The only response we received was from Tanzella of SRI.
"I can't speak on the record about a report that was primarily written by McKubre," Tanzella wrote.
As we went to press, McKubre had not responded to our inquiry.
The SRI "Case" Replication
In 1998, researchers at SRI International performed the Case replication experiment, named after its inventor, Lester Case. This LENR experiment resulted in one of the most important findings of the field: a clear relationship between the measurements of excess heat and 4He. This helped to establish the authenticity of the nuclear source of excess heat and helium-4 in LENR experiments.
The Case experiment was not an electrolytic experiment with heavy water but a deuterium gas and palladium-on-carbon catalyst configuration. It produced a rigorous result of 32 Mev/4He. Only one little problem: The subgroup of LENR researchers had predicted that the experiment would produce ~24MeV/4He on the basis of the hypothesized D+D —> 4He + ~24MeV/4He (heat) “cold fusion” reaction.
Had this experiment shown a value that was ~24Mev/4He, this would have helped to validate the subgroup's theories that LENR is a "cold fusion" process. Instead, the Case replication disproved them.
The authors of the 2000/2004 papers, not willing to accept defeat easily, decided to devise an explanation for the experiment’s not matching their D-D "cold fusion" idea.
So they figured out a way to use the results of M4, an electrolytic experiment that took place in 1994, to explain the results of the Case gas experiment, which took place in 1998.
The SRI and MIT researchers in the 2000/2004 papers, without stating they had done so, developed new interpretations and explanations about the 1994 experiment. With these in hand, in a two-step process, they first claimed that the 1994 experiment did, in fact, produce ~24 MeV/4He.
Then, they claimed that this newly interpreted ~24 MeV value for the 1994 experiment, in conjunction with the helium retention hypothesis, could explain the large deviation of 32MeV from ~24MeV that was observed in the 1998 Case experiment.
When the researchers described the two experiments in the 2000 paper, they reversed the order (1998 listed as No. 2, 1994 listed as No. 3) and provided text implying that their 1994 experiment took place after the 1998 experiment. Readers were led to believe that the earlier experiment represented added confirmation for the latter experiment.
Helium Is a Noble Gas - It Does Not Dissolve Into Palladium
The helium retention hypothesis has two more problems.
The first problem is that helium does not dissolve into a metal or get absorbed directly into an intact metallic lattice structure, as do hydrogen isotopes, which form metallic hydrides.
More than a century ago, William Ramsay, Fellow of the Royal Society, and Morris W. Travers published "An Attempt to Cause Helium or Argon to Pass Through Red-Hot Palladium, Platinum or Iron." [5]
"Neither argon nor helium is able to pass through any one of these metals, even at fairly high temperatures," Ramsay and Travers wrote.
The second problem with the helium retention hypothesis is that, because helium isotopes do not dissolve in metals, they can move through metallic structures only in voids or cracks or grain boundaries of micro- or macro-scales. Thus, imperfect metallic structures can exhibit high permeability to helium isotopes, which assures rapid release of helium, especially in cases of thermal-mechanical disturbances that occur on the surfaces of palladium cathodes in electrolytic or gas-phase cells. [6]
Evidence for such thermal-mechanical activity can be seen by SEM imaging in palladium cathodes used in LENR experiments. Surfaces of the cathodes show many features, such as craters, deformations and hot spots, where the palladium surface has either melted or explosively changed. The melting point of palladium is 1555 C, which is about 30% of the surface temperature of the sun.
Other evidence for this surface activity appears in depth-profiling of such cathodes with mass spectroscopy, which reveals that transmutation products invariably occur only within several thousand angstroms of the cathode surface.
During the course of LENR experiments, significant amounts of material are typically removed from the surface of the cathode. Such thermal-mechanical removal and destruction of the surface of the metallic lattice that forms the cathode would insure rapid release of any helium isotopes temporarily trapped within the near-surface regions.
This explains why Danielle Gozzi, a professor of physical chemistry with the University of Rome, found no detectable helium even when he melted and analyzed sections of the Pd cathode. [7]
And the authors of [3] knew that helium would permeate surface defects when they made the claim of helium retention to the Department of Energy.
On page 24 in their paper presented to the DoE, they attempted to explain a "discrepancy relative to the integrated excess heat" and provided a possible explanation: "The bulk of product helium was lost before sample collection due to micro-fractures, which occur in surface-stressed Pd, as described by A. Farkas." [8]
Comment on this article.
Acknowledgements
The author wishes to acknowledge the prior work of two people who have published important discussions relating to helium-4 in LENR experiments.
The first is analyst Jeffrey Hullekes, for his "Rethinking Reactants" paper submitted to but not presented at the University of Missouri LENR Symposium on May 29, 2009.
The second is Lewis Larsen, who conceived the Widom-Larsen theory of LENRs, also known as the ultra-low momentum nuclear reaction theory. Larsen published ideas about helium-4 in LENR experiments in a Sept. 3, 2009, Web-published slide presentation, which explores the Case replication experiment.
References
1. Development of Energy Production Systems from Heat Produced in Deuterated Metals - Energy Production Processes in Deuterated Metals, Volume 1, TR-107843-V1, Thomas Passell (Project Manager,) Michael McKubre, Steven Crouch-Baker, A. Huaser, N. Jevtic, S.I. Smedley, Francis Tanzella, M. Williams, S. Wing (Principal Investigators,) B. Bush, F. McMohon, M. Srinivasan, A. Wark, D. Warren (Non-SRI Contributors,) June 1998
2. Michael McKubre, Francis Tanzella, Paolo Tripodi and Peter Hagelstein, "The Emergence of a Coherent Explanation for Anomalies Observed in D/Pd and H/Pd Systems; Evidence for 4He and 3He Production" 8th International Conference on Cold Fusion. 2000. Lerici (La Spezia), Italy: Italian Physical Society, Bologna, Italy.
3. Peter Hagelstein, Michael McKubre, David Nagel, Talbot Chubb, Randy Hekman, "New Physical Effects In Metal Deuterides," Submitted to the 2004 U.S. Department of Energy LENR Review
4. Hagelstein, P.L., Summary of ICCF3 in Nagoya, Feb. 16, 1993. 1993.
5. Ramsay, W.; Travers, M.W. "An Attempt to Cause Helium or Argon to Pass through Red-Hot Palladium, Platinum, or Iron." Proceedings of the Royal Society of London (1854-1905). 61 (-1), p. 266-267. Jan. 1897, doi:10.1098/rspl.1897.0034
6. Schultheis, D., "Permeation Barrier for Lightweight Liquid Hydrogen Tanks," Ph. D. thesis, University of Augsburg (Germany), 2007
7. Gozzi, D., et al., "X-Ray, Heat Excess and 4He in the D:Pd System," Journal of Electroanalytical Chemistry, Vol. 452, p. 253, (1998) and Erratum, 452, 251–271, (1998)
⇐ Previous Article — Table of Contents — Next Article ⇒ |